Cable-like smart matter |
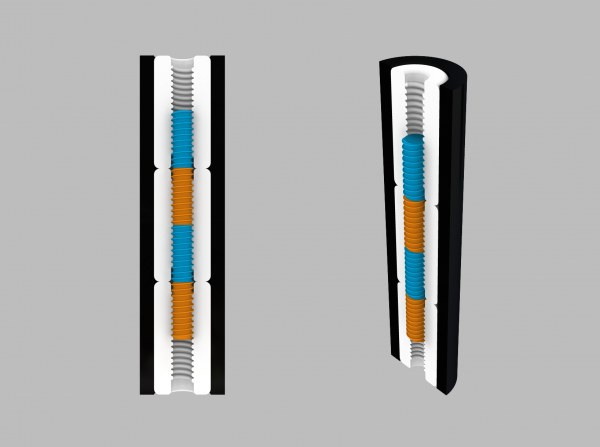 |
A typical Ouro filament or nema |
Ouro is a polymorphic material architecture that is based on swarm robotic filaments that join together to form a variety of arrays with different properties.
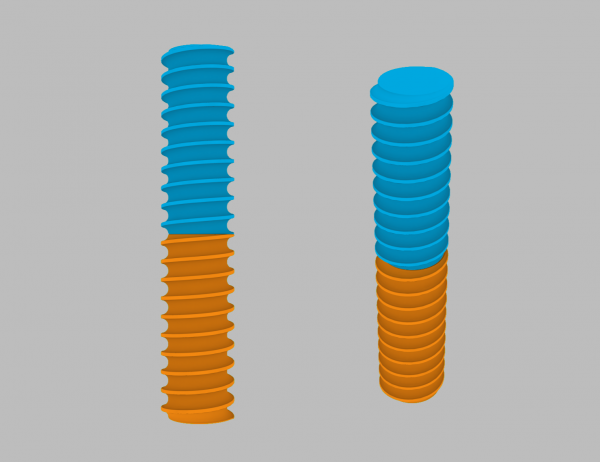 |
The internal screw mechanism of an ouro filament |
An individual ouro filament, known as a nema[1], consists of three primary elements: a series of thick-walled hollow cylinders, flexible bi-screw mechanisms, and a flexible muscular sleeve. The three elements work together to create a structure that can vary its stiffness across a gradient from completely flexible to rigid.
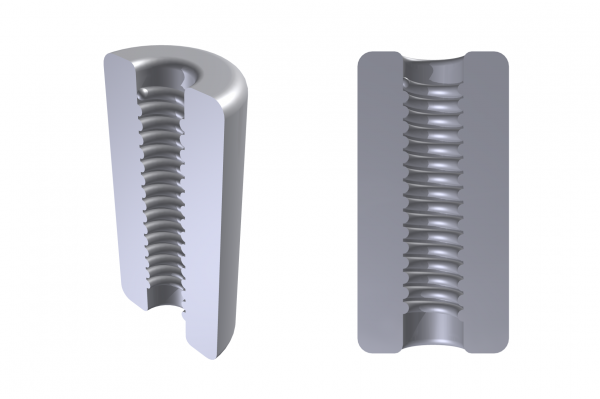 |
Two views of a cylinder that has been cut to show the inside |
By contracting along its long-axis, the muscular sleeve decreases the distance between the cylinders. Full contraction puts the cylinder series under compression. Simultaneously to the muscular sleeve's contraction, the bi-screws' screws contra-rotate in the cylinders' threaded holes--this action draws the cylinders together. The bi-screws maintain the distance between the cylinders or their compression loading after the muscular sleeve relaxes.
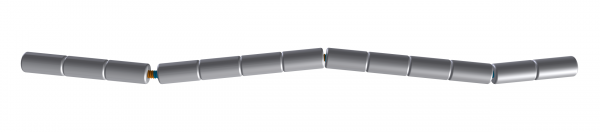 |
A section of a nema displaying how semi-rigidity is achieved. |
The nema's stiffness is adjustable by varying the number of cylinders that are screwed tight against one another, and the distance between the cylinders that aren't. The greater the number of gaps between cylinders and/or the gaps' size, the more flexible the nema becomes.
In ultratech ouro, the muscular sleeves are composed of aggregated linear nano-electrostatic motors. In transapient-tech ouro, the sleeves are composed of ultimate muscle.
The muscular sleeve has a PQDA-equipped outer skin that performs several functions. The PQDA creates electrically conductive patches that allow nemata to transmit power and data to one another through contact. It allows nemata to vary the coefficient of friction of their skin, becoming slippery or gripping; this aids the nemata when they are locomoting. Finally, it allows bulk ouro to vary its surface properties--such as functioning as an optical phased array display or imaging sensor. Transapient-tech ouro additionally often employs quantum impeller technology in the skin to allow the nemata to slide past one another with much greater speed and virtually without friction.
Depending on the specific design, the cylinders and/or the bi-screw mechanisms contain the nema's computers and energy storage elements. Energy storage methods vary according to design, and include nanoflywheels and supercapacitors. In many ouro designs the cylinders are composed of densely 3D-woven PQDA nanofibers. The cylinders can therefore generate computers and capacitors within them.
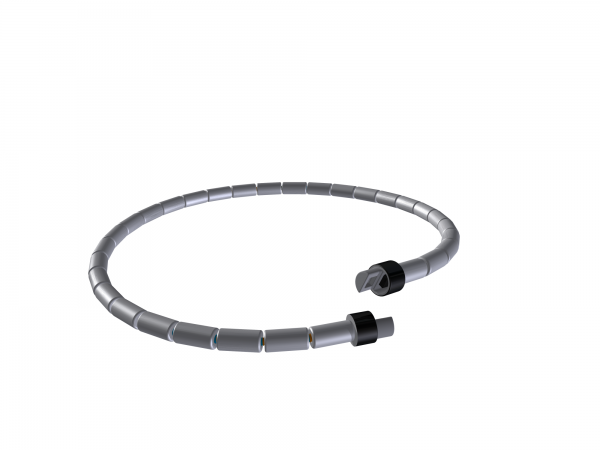 |
Ring formation in an ouro thread. |
Nemata join together into ouro using three fundamental methods. The first is ring-weaving. In ring-weaving, nemata use their hermaphroditic couplers to form into closed rings. The rings interlink with other rings to form maille. Maille weaves may be single layer quasi-2D sheets, or 3D-weaves that can form bulk solids. Different maille weaves have different properties. Some 3D-weaves produce rigid materials, other 3D-weaves result in semi-rigid materials. Quasi-2D weaves produce flexible maille sheets.
In the second fundamental method, nemata join end-to-end to form long fibers. These fibers self-weave or braid into quasi-2D fabrics, cords, or 3D bulk materials.
In the third fundamental method, nemata join together using supplementary nodes. Nodes are spherical, and have hermaphroditic couplers arrayed on their surface, typically with Platonic polyhedral symmetry. Nodes allow ouro to form space-frame materials with a millimeter scale truss structure.
Except for in its space-frame architecture, ouro is a densely interconnected material. This property makes it one of the toughest of the polymorphic materials. Because of its dense interconnectivity and the methods that nemata use to locomote, ouro cannot reconfigure itself nearly as rapidly as utility fog.
Ouro is one of the technologies used to produce gelbots. Ouro fabric is used in some fancloth designs, roll-up aircraft wings, self-deploying packaged buildings, and strength-augmenting clothing. Braided ouro ropes can act as robotic tentacles that can become rigid. Multi kilometer long spooled ouro ropes are used as push/pull/torque tractor-lines in zero gee. Liquid or gas-delivery hoses sleeved in ouro can move themselves into position.
Space-frame ouro is similar to utility fog in some respects. It can flow rapidly, but lacking telescopic elements, it cannot change its volume while maintaining the omni-triangulated architecture that gives u-fog its structural strength.
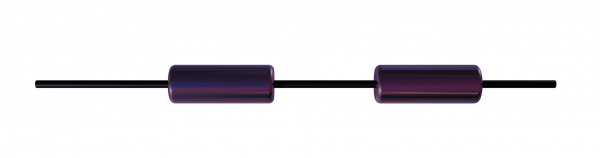 |
Ouro bead thread configuration |
Ouro can form hybrid materials with other compatible technologies. If they have the same hermaphroditic coupler design, operate on the same power standard, and use compatible software, nemata can link together with utility foglets. Beads with thru holes or grains with eyelets can be strung on long ouro threads. Beads and grains range from sub-millimeter to macroscale, and can contain technologies that are too large to fit inside nemata, such as nanoforges, sensors like olfactory arrays, synsect drones, stowed geckotech feet or bushbot manipulators, tool boxes, and fold-up motorized wheels.
On its own, ouro is not impermeable to gases or liquids. If it is hybridized with platelet-bots[2], ouro can be used to construct polymorphic spacecraft hulls, aerostatic craft, aquatic-craft hulls, large pneumatically-supported fabric buildings and other structures, shape-changing, self-healing pneumatic tires; MPTCs; and adaptive plumbing. Ouro can be water-resistant on its own if the specific weave is tight enough and the PQDA skin generates a hydrophobic surface; sufficient water pressure will compromise the resistance by forcing its way through the interstices of the weave.
Ouro with a high PQDA content can, in macroscale amounts, form electromagnets with significant field strengths. This allows bulk masses of ouro to magnetically levitate over other ouro or specialized maglev systems. Levitating ouro is powered by beamed energy. Lasers or electro-lasers generated by nemata's PQDA skin transmit energy to receivers generated in the surface of the levitating mass.
Nemata come in different scale sizes. The smallest are 20 micrometers in diameter and about 425 micrometers long. One of the most common sizes is 40 micrometers in diameter and 1.7 millimeters long.
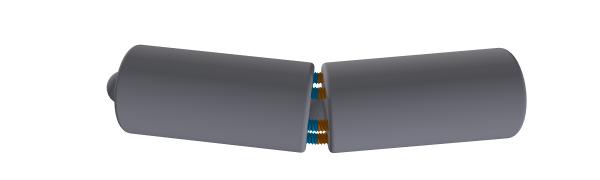 |
BAS (Ball-and-socket) ouro |
There are a number of nema variants beyond the baseline architecture. The most common of these, the BAS, utilizes ball-and-socket joints on the cylinders, and six or more bi-screws in each cylinder end. The advantage of this design is that the nema can remain rigid while it is curved and passive. This is possible because the bi-screws maintain the cylinders under compression, thru the ball-and-socket joint, at all flexural angles.
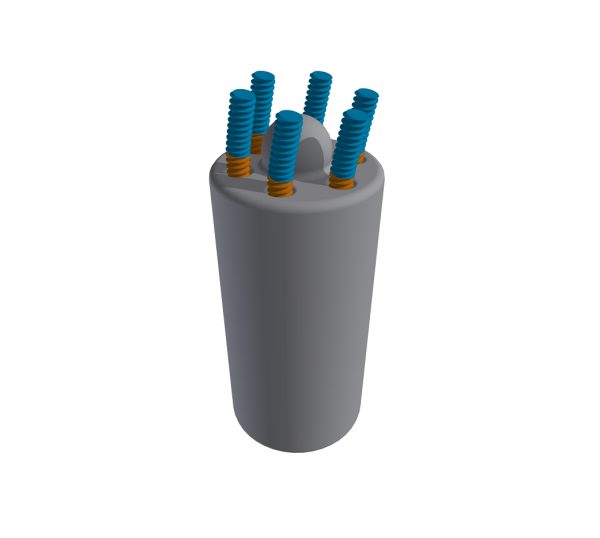 |
BAS ouro, showing the ball at one end |
BAS can become passively flexible or semi-rigid if the bi-screws extend in unison far enough to unseat the ball from the socket. BAS is the nema design most often used in gelbots.
Some macroscale nema designs have numerous legs. These legs have the same basic structure as baseline nemata. If the legs are arrayed radially, the legged nemata resemble hairy worms. Some are bilaterally symmetrical; the legs are arrayed in rows down the sides. The legs are typically tipped in hermaphroditic couplers and/or micro-barbs, claws, pincers, or geckotech setae.
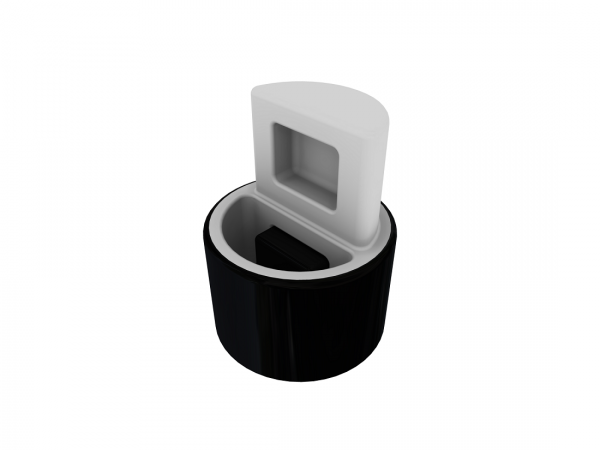 |
The hermaphroditic coupler at each end of an ouro nema |
[1] 'Nema' is the Greek word for thread. The plural is nemata. back
[2] Platelet-bots are flat, flexible, mobile, swarm-robotic tiles. They can reversibly adhere to one another to form membranes or rubbery solids that are impermeable to gases and/or liquids. back
The name ouro is derived from ouroboros, the symbol of a snake eating its tail.